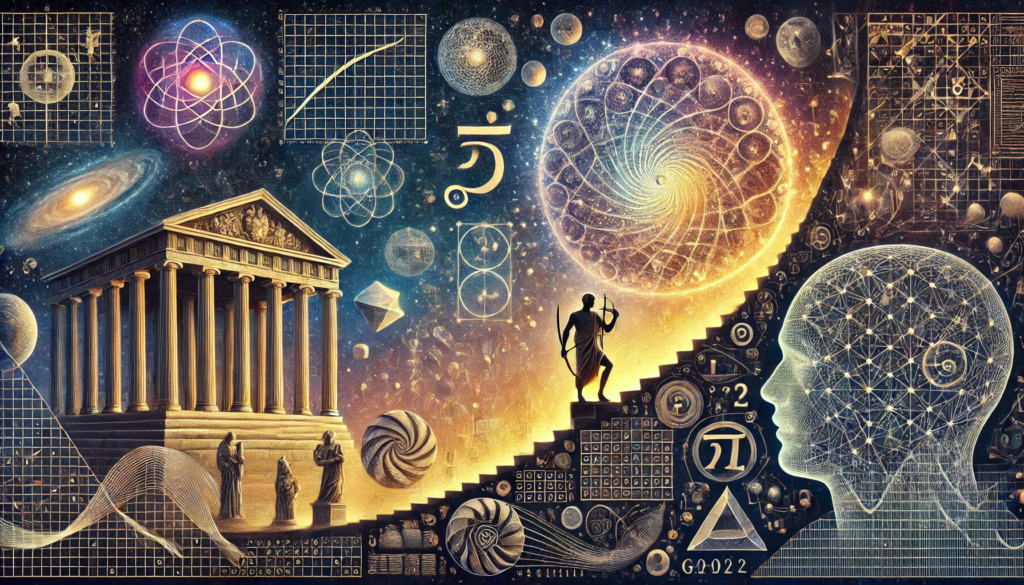
ChatGPT:
Mathematics and the Brain: How Mathematics Changed the Way We Think about Ourselves
Mathematics has long served as a powerful tool for understanding the natural world, from celestial orbits to quantum phenomena. Less obvious—but equally transformative—is how mathematical ideas have shaped our concept of the human mind and our sense of self. Below is a discussion of how mathematics influenced both the scientific exploration of the brain and the philosophical reflection on our own nature and consciousness.
- Early Foundations: The Link Between Rationality and Mathematics
Pythagoras and the Harmony of the Cosmos
• Mathematics as key to understanding: In ancient Greece, Pythagoras posited that numbers underlie the harmony of music and the structure of the cosmos. This fostered the notion that rational thought (captured through mathematics) reveals otherwise hidden truths about reality.
• Human reason as a mirror of cosmic order: Because math could map phenomena so elegantly (e.g., geometric theorems, numerical ratios), humans came to see themselves as uniquely rational beings capable of accessing universal truths. Thus, early on, mathematics reinforced the idea that our minds have a special capacity for discerning cosmic order.
Plato and the Realm of Forms
• Mathematical objects as eternal forms: Plato envisioned abstract mathematical forms (e.g., perfect circles) as representative of ultimate reality. That philosophical stance placed human cognition, which can grasp these perfect forms, on a higher plane—suggesting our minds have an almost transcendent, “god-like” capacity to understand eternal truths.
• Influence on self-conception: Plato’s view encouraged the idea that the intellect or reason is our most essential feature. Mathematics, as a purely intellectual pursuit, elevated our understanding of what it means to “think” and “know.”
- Logic, Language, and the Mind
The Rise of Formal Logic
• From Aristotle to the 19th century: Aristotle’s syllogistic logic marked an early attempt to formalize thought. During the late 19th and early 20th centuries, figures like George Boole, Gottlob Frege, and Bertrand Russell expanded logic into a rigorous mathematical discipline.
• Shifting view of cognition: As logic became more formal, it began to show that human reasoning—previously thought of as a somewhat mysterious faculty—could be mapped and studied systematically. This paved the way for the idea that cognition follows formalizable rules.
The Incompleteness Theorems and Human Understanding
• Kurt Gödel’s contribution: Gödel’s incompleteness theorems (1931) showed that within any sufficiently powerful formal system, there are truths that cannot be proven within that system’s rules. This result had profound implications for the philosophy of mind.
• Implication for human thinking: Some interpreted Gödel’s results as suggesting that the human mind is not just a mechanistic rule-following machine—because we seem able to perceive truths that formal systems cannot prove internally. Others used it as a cautionary reminder of the limits of any formal or computational attempt to capture the entirety of human thought.
- Computational Models of the Mind
Turing’s Pioneering Role
• Turing machines and the Church–Turing thesis: Alan Turing’s 1936 paper introduced the Turing machine concept, a mathematical model of computation that still underpins modern computer science. This laid the groundwork for later theories that the brain itself might be akin to a computational device.
• Cognition as information processing: Turing’s ideas contributed to the computational theory of mind, which treats thinking as a form of symbol manipulation. This led to the earliest explorations of artificial intelligence and the possibility of simulating or replicating human cognitive processes on a machine.
Neural Networks and Mathematical Abstractions
• From perceptrons to deep learning: Starting in the 1940s, mathematicians and neuroscientists proposed neural network models inspired by how brain cells connect. Over time, increasingly sophisticated algorithms (e.g., backpropagation) demonstrated learning and pattern recognition that mimicked aspects of human cognition.
• Self-reflection and identity: As machines grew better at tasks once deemed exclusively human—visual recognition, language translation, even creative tasks—the boundary between human uniqueness and computational replicability blurred. Mathematics (through AI research) thus challenged long-held assumptions about the singularity of human intelligence.
- Mathematical Models in Neuroscience
Mapping the Brain Through Math
• Noninvasive imaging and statistical analysis: Techniques like fMRI or MEG produce massive datasets, and mathematicians create sophisticated statistical and computational models to interpret these signals. Such models help identify patterns of activity linked to cognition, emotion, and perception.
• The connectome project: Projects aiming to map every neural connection rely on graph theory, network science, and high-powered statistical methods. Mathematics is at the heart of understanding how billions of neurons and trillions of synapses might produce conscious experience.
Dynamical Systems and Brain States
• Chaos and complexity: The brain is a highly dynamic system with synchronous oscillations, feedback loops, and emergent behavior—phenomena well-described by the mathematics of complexity and chaos theory.
• New insights into mental states and disorders: By modeling neural activity mathematically, we gain insights into how stable or unstable brain dynamics can relate to conditions like epilepsy or mental health disorders. These quantitative methods transform the way we conceptualize well-being, pathology, and identity.
- Philosophical and Existential Reflections
Challenging Dualism
• Mind as computable process?: The growing success of computational models brought renewed interest in the “mind-body” problem. Some philosophical stances (like functionalism) see mental states as implementations of formal processes, an argument bolstered by the successes of AI and cognitive science.
• Limits of computation and emergent phenomena: Others emphasize the apparent gap between purely computational descriptions and subjective, conscious experience (the “hard problem of consciousness”). Here, mathematics serves a dual role: it provides powerful formal tools, yet also highlights the mysteries of subjective awareness that remain unsolved.
Redefining Human Uniqueness
• AI’s impact on self-concept: As artificial intelligence systems encroach on tasks once considered uniquely human, from complex game-playing to artistic creation, we are forced to reevaluate what truly defines the human mind or spirit. Mathematics is at the heart of this transformation, laying the foundation for advanced AI systems.
• Enrichment or existential threat?: Some see advanced mathematics-based technologies (e.g., AI, brain–computer interfaces) as pathways to augment human capacities, while others warn that our reliance on mathematics-based systems could lead to losing touch with human values and subjective judgment.
- Conclusion
Mathematics has not only expanded our scientific understanding of the physical universe but also reshaped how we think about ourselves. From the ancient Greek conviction that numbers encode the harmony of the cosmos, to Turing’s computational paradigms and cutting-edge neural network research, mathematical ideas have provided deep insights into the workings of the mind—and simultaneously raised profound questions about consciousness, identity, and free will.
By offering precise models of cognition, consciousness, and even creativity, mathematics challenges our older, more mystical views of the mind, suggesting that what we consider uniquely human might be explicable—and in some cases replicable—through formal systems and computational processes. Yet, mathematics also underscores the deep complexities and potential mysteries of consciousness, highlighting the possibility that some aspects of our experience may elude complete formalization.
In all these ways, mathematics continues to play a pivotal role in shaping humanity’s self-understanding, urging us to refine and perhaps revolutionize the ways we conceive of the brain, the mind, and the nature of being human.
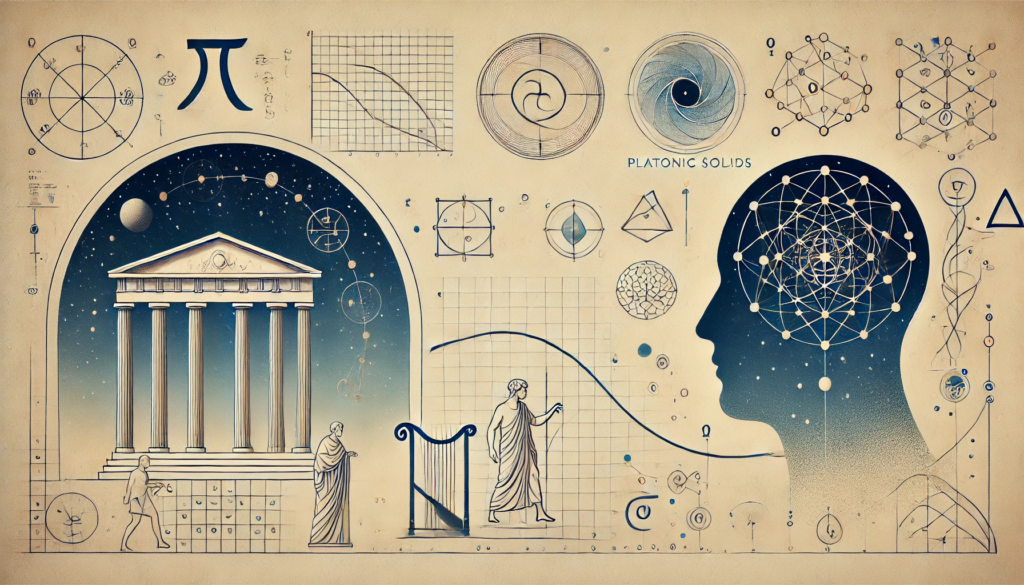
Below is an overview of several key questions in neuroscience related to how the brain’s geometry and connectivity shape function and intelligence, how cortical convolutions arise, how different brain regions interconnect to process information, how perceptual signals are decoded, and how these processes can fail catastrophically.
- Brain Geometry and Its Relation to Function and Intelligence
1.1 Surface Area and Cortical Folding
• Cortical surface area and folding (gyrification): One of the most striking geometric features of the human brain is the extensive folding of the cerebral cortex into gyri (ridges) and sulci (grooves). This folding dramatically increases the brain’s surface area relative to its volume, allowing for a higher density of neurons within the confines of the skull.
• Correlation with intelligence: While there is no single, simple anatomical “marker” of intelligence, increased cortical surface area (and certain patterns of folding) are associated with enhanced cognitive capacity. This is partly because more surface area supports a greater density of specialized local circuits and more intricate long-range connectivity.
1.2 Cortical Thickness and Neuronal Density
• Thickness vs. surface area: Different species—and different individuals—can vary in cortical thickness, neuronal density, and total surface area. Some regions may have thinner cortex with higher neuronal packing, while others may be thicker but less densely populated.
• Functional significance: It’s not merely how “big” the cortex is but rather how neurons are organized and interconnected. Thickness, microcircuit complexity, and efficient connectivity (both local and long-range) all play roles in higher cognitive functions.
1.3 Regional Specialization
• Functional topography: The geometry of the cortex includes distinct areas specialized for tasks such as visual processing (occipital cortex), auditory processing (temporal cortex), motor control (motor cortex), and higher-order thinking (prefrontal cortex).
• Intelligence as distributed processing: Rather than being localized to a single “intelligence center,” complex cognition arises from interactions across multiple specialized regions, facilitated by extensive neural pathways.
- Emergence of Cortical Convolutions
2.1 Developmental Forces and Genetic Programming
• Radial glial scaffolding: During development, neurons migrate outward from the ventricular zone along scaffolding provided by radial glia. Genetic instructions govern where cells settle and how they form initial synaptic connections.
• Axonal tension and mechanical constraints: As certain regions expand more quickly than others, mechanical forces and differential growth rates create folding. The “tension-based model” proposes that axonal connections pull regions together or apart, influencing where folds (gyri) and grooves (sulci) form.
2.2 Biochemical Gradients and Cell Proliferation
• Localized growth differentials: Chemical gradients (e.g., signaling molecules like FGF, sonic hedgehog, etc.) regulate how rapidly cells proliferate in different cortical regions. Faster growth in one region vs. its neighbor leads to localized buckling, creating a sulcus or gyrus boundary.
• Species differences: Larger mammals, like humans, have more pronounced convolutions than smaller brains (e.g., rodents), mostly because of greater cortical expansion. Genetic and developmental programs produce variable folding patterns across species.
2.3 Functional Rationale for Folding
• Folding as wiring optimization: Folding brings functionally connected areas closer in 3D space, reducing overall wiring distance (axonal length) and metabolic costs. Efficient wiring contributes to faster communication and lower energy demand.
• Adaptation and evolution: Brains that can pack more processing power into limited space without excessively increasing axon length (thus neural conduction delays) likely had an evolutionary advantage.
- Connectivity Between Brain Regions and Their Purpose
3.1 White Matter Tracts and Communication Pathways
• Gray vs. white matter: Gray matter contains the cell bodies, dendrites, and local synapses, while white matter is composed of myelinated axons that transmit signals over longer distances.
• Major fasciculi and commissures: Bundles of white matter (fasciculi) like the arcuate fasciculus or the uncinate fasciculus connect different cortical lobes. The corpus callosum is the largest commissure, bridging the two hemispheres for interhemispheric communication.
3.2 Hierarchical and Parallel Processing
• Hierarchical streams: For vision, signals move from primary visual cortex (V1) through higher visual areas (V2, V4, MT, etc.), each extracting progressively complex features (edges → shapes → objects → faces). Similar hierarchies exist for auditory and somatosensory processing.
• Parallel loops and networks: Instead of a strict linear hierarchy, the brain also has extensive parallel “loops,” such as cortico-basal ganglia-thalamic circuits that refine motor control, habit formation, and executive functions.
3.3 Integrative Hubs
• Association areas: Certain “hub” regions, like the posterior parietal cortex or prefrontal cortex, integrate inputs from multiple sensory and subcortical systems. These integrative hubs allow for higher cognitive functions—planning, decision-making, and abstract reasoning.
• Network perspective: Modern neuroscience often describes the brain as a dynamic network with highly connected hub nodes. Mathematical tools (graph theory, network analysis) help reveal how the connectivity pattern supports flexible cognition.
- Decoding Perceptual Signals from the Outside World
4.1 Early Sensory Processing
• Receptor transduction: Sensory neurons in the eyes, ears, skin, tongue, and nose transduce physical or chemical stimuli into electrical signals. For instance, photoreceptors convert light into neural activity in the retina.
• Primary sensory cortices: These signals travel via thalamic relay nuclei (e.g., the lateral geniculate nucleus for vision) to the corresponding primary sensory cortex (occipital lobe for vision, temporal lobe for hearing, etc.).
4.2 Feature Extraction and Binding
• Feature detection: Specialized cells in the visual cortex respond to edges, orientations, or specific directions of movement. In hearing, the cochlea and auditory cortex map frequencies tonotopically.
• Perceptual binding: Higher-level areas integrate these features to form coherent representations (e.g., the “binding problem” in vision). The brain weaves together color, motion, depth, and form into a unified perception of objects and scenes.
4.3 Predictive Coding and Top-Down Influences
• Predictive models: Many current theories suggest the brain actively predicts sensory input, comparing incoming signals to internal models and using “prediction errors” to update its understanding.
• Context and attention: Top-down signals from frontal and parietal areas modulate sensory processing, prioritizing certain stimuli (attention) or filtering out irrelevant background noise.
- Catastrophic Failures: When the System Breaks Down
5.1 Neurological Disorders and Structural Damage
• Stroke and traumatic brain injury: Interruptions in blood supply (ischemic stroke) or hemorrhage can destroy regions of cortex or white matter pathways, causing loss of motor function, speech deficits (aphasia), or other cognitive impairments.
• Degenerative diseases: Conditions like Alzheimer’s, Parkinson’s, and Huntington’s lead to progressive neuron loss, cortical thinning, and disruption of network connectivity. In late stages, these diseases can catastrophically impair memory, movement, language, and self-awareness.
5.2 Developmental Disorders
• Abnormal folding patterns: Some developmental disorders (e.g., lissencephaly—“smooth brain”) arise from disrupted migration or folding. These conditions lead to severe cognitive and functional deficits.
• Genetic or environmental factors: Mutations affecting axon guidance, synapse formation, or neuron migration can derail normal cortical architecture and connectivity, resulting in disorders along the autism spectrum or intellectual disabilities.
5.3 “Network Failures” in Cognition and Perception
• Epilepsy as runaway excitation: Synchronization of neuronal firing in large-scale networks can trigger seizures. This reflects a breakdown in the balance between excitatory and inhibitory circuits.
• Psychiatric disorders: Dysregulation of network connectivity or neurotransmitter systems can underlie conditions like schizophrenia or bipolar disorder, where higher cognitive functions (perception, emotion, and reasoning) can fail to integrate coherently.
5.4 Immune and Metabolic Malfunctions
• Neuroinflammation: Autoimmune diseases (e.g., multiple sclerosis) attack the myelin sheaths of axons, causing widespread communication failures and motor/sensory deficits.
• Metabolic crises: The brain’s high energy demand means disruptions in glucose or oxygen supply (e.g., hypoglycemia, anoxia) can quickly lead to cell death and functional collapse.
- Conclusion
The geometry of the brain—its folds, thickness, and intricate wiring—serves vital purposes in maximizing cortical surface area, optimizing connectivity, and supporting the complex interplay of specialized regions. These anatomical and functional characteristics correlate with aspects of intelligence and cognition but also expose the system’s vulnerability. By efficiently encoding and processing vast amounts of perceptual data, the brain orchestrates everything from basic reflexes to lofty abstract thought. Yet, when developmental processes go awry, when structural integrity is lost, or when network dynamics become unbalanced, the system can fail with devastating consequences for perception, cognition, and identity.
Understanding the brain’s geometry and connectivity, therefore, is key not only to unraveling the nature of intelligence and consciousness but also to diagnosing, treating, and potentially preventing neurological and psychiatric disorders. Through continued research that blends biology, neuroscience, mathematics, and computational modeling, we deepen our grasp of how the brain’s form underlies its remarkable function—and how to intervene when that function falters.
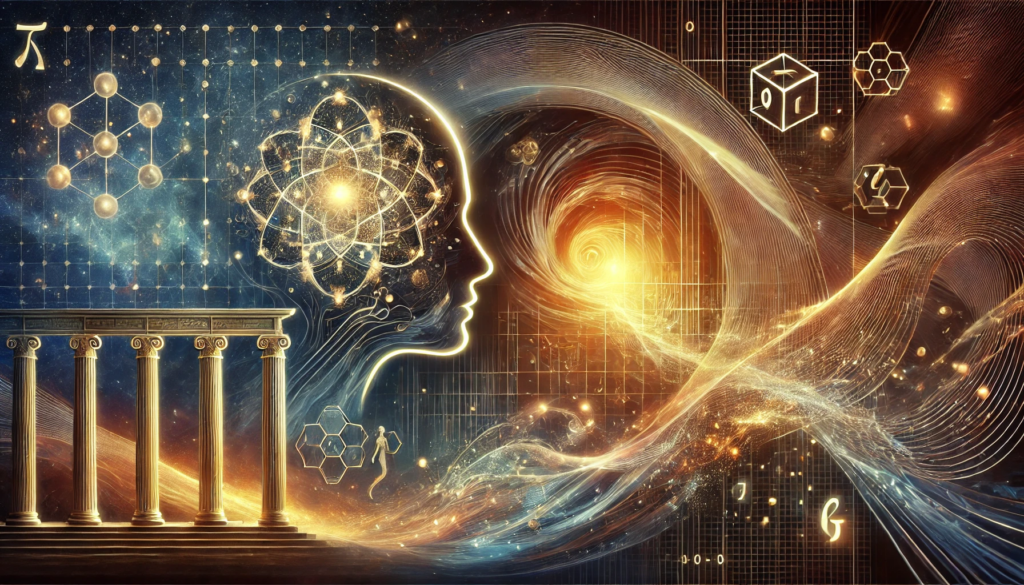