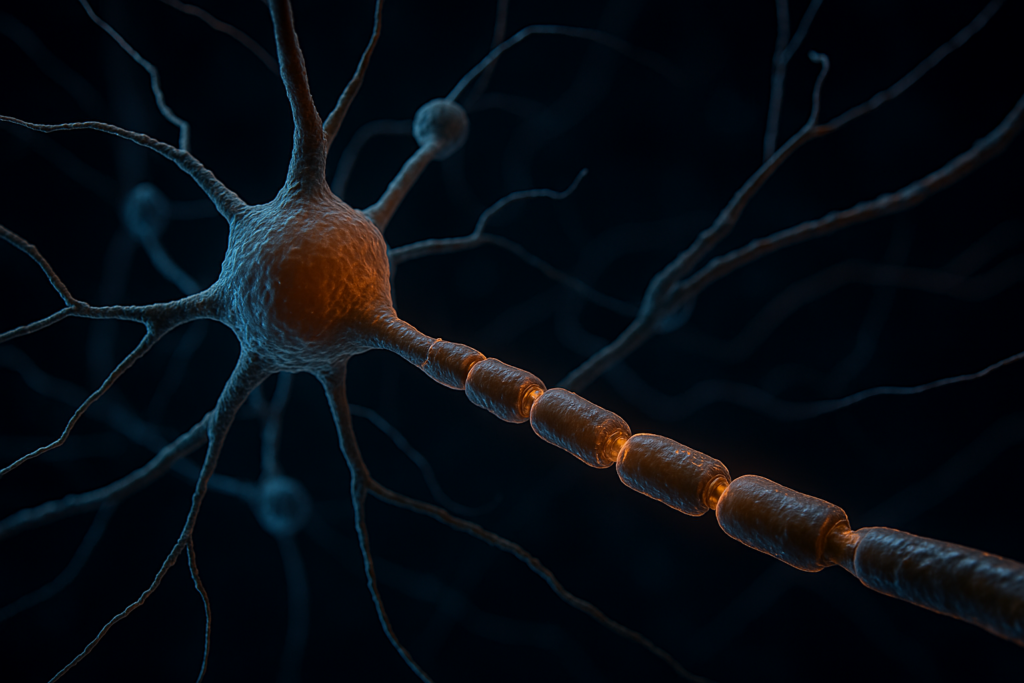
ChatGPT:
The Unsung Heroes of the Nervous System: Glia, Myelin, and
Axons in Brain Function
Introduction
The human brain, a marvel of biological engineering, is composed of an intricate network
of cells that work in synchrony to generate thought, sensation, movement, memory, and
emotion. While neurons-the primary signaling units-have long dominated the spotlight in
neuroscience, another class of cells, called glial cells, are increasingly recognized as
essential contributors to brain function. Once dismissed as mere support cells, glia are
now known to play active, dynamic roles in maintaining br…
1. Glial Cells: An Overview
Glial cells, or neuroglia, are non-neuronal cells in the central and peripheral nervous
systems. They do not conduct electrical impulses like neurons, but they are essential for
maintaining a stable environment, providing support and nutrients, and insulating and
protecting neurons. Glial cells outnumber neurons in the human brain, suggesting their
significance has been vastly underestimated in traditional neuroscience narratives.
There are several main types of glial cells, each with specialized functions: astrocytes,
oligodendrocytes, microglia, Schwann cells, and ependymal cells. These cells are found
in different parts of the nervous system and contribute uniquely to neural operations.
Astrocytes are star-shaped cells that support the blood-brain barrier, regulate blood flow,
provide nutrients to neurons, and help maintain the chemical environment around
synapses. Oligodendrocytes are responsible for myelinating axons in the central nervous
system, while Schwann cells perform the same function in the peripheral nervous system.
Microglia act as immune cells, removing waste and responding to injury or infection.
Ependymal cells line the brain’s ventricles and help circulate cerebrosp…
2. Functions of Glial Cells
Beyond their traditional role as support cells, glial cells are actively involved in modulatingsynaptic activity and shaping neural communication. This understanding marks a
significant shift in how scientists perceive brain function.
Astrocytes, for example, are part of the “tripartite synapse,” a concept that includes the
pre-synaptic neuron, the post-synaptic neuron, and the astrocyte. These glial cells can
detect neurotransmitter levels and release their own signaling molecules, called
gliotransmitters, to modulate synaptic strength and plasticity. This places glia squarely in
the realm of information processing.
Oligodendrocytes and Schwann cells, through myelination, increase the speed of action
potential propagation, which enhances communication between different parts of the
nervous system. Meanwhile, microglia monitor the brain for signs of damage or infection
and play a crucial role in synaptic pruning-removing unnecessary or weak synapses
during development and disease.
The multifaceted functions of glial cells illustrate their central role in maintaining neural
circuits, enabling learning, memory, and adaptation.
3. The Axon: Pathway of Neural Communication
The axon is a long, slender projection of a neuron that transmits electrical signals from
the cell body to other neurons or target tissues. Each neuron has a single axon, which
can branch extensively to form connections across various regions.
Signal transmission along the axon is facilitated by the movement of ions across the
neuronal membrane, a process that generates an electrical signal called an action
potential. This signal originates at the axon hillock and travels toward the axon terminals,
where it triggers the release of neurotransmitters.
Axons can be either myelinated or unmyelinated. Myelinated axons are wrapped in a fatty
insulating substance called myelin, which allows for saltatory conduction-where action
potentials jump from one Node of Ranvier to the next. This significantly increases the
speed and efficiency of signal transmission, often up to 100 meters per second.
Unmyelinated axons conduct impulses more slowly, as the action potential must travelcontinuously along the membrane. These fibers are typically involved in slower, less
urgent types of communication, such as dull pain or temperature regulation.
4. Myelin Sheath: Enhancing Signal Speed and Efficiency
The myelin sheath is composed primarily of lipids and proteins and is produced by
oligodendrocytes in the CNS and Schwann cells in the PNS. It wraps around axons in
segments, with small gaps called Nodes of Ranvier.
Myelin serves several crucial functions. It acts as an electrical insulator, preventing signal
loss and enabling saltatory conduction. This allows electrical impulses to travel much
faster and with less energy consumption. Myelin also protects axons from physical
damage and provides metabolic support.
Damage to the myelin sheath can have devastating effects. Diseases like multiple
sclerosis involve immune-mediated destruction of myelin, leading to impaired signal
conduction, muscle weakness, sensory disturbances, and cognitive decline. The
regeneration of myelin is an active area of research, with therapies aimed at promoting
remyelination and restoring neural function.
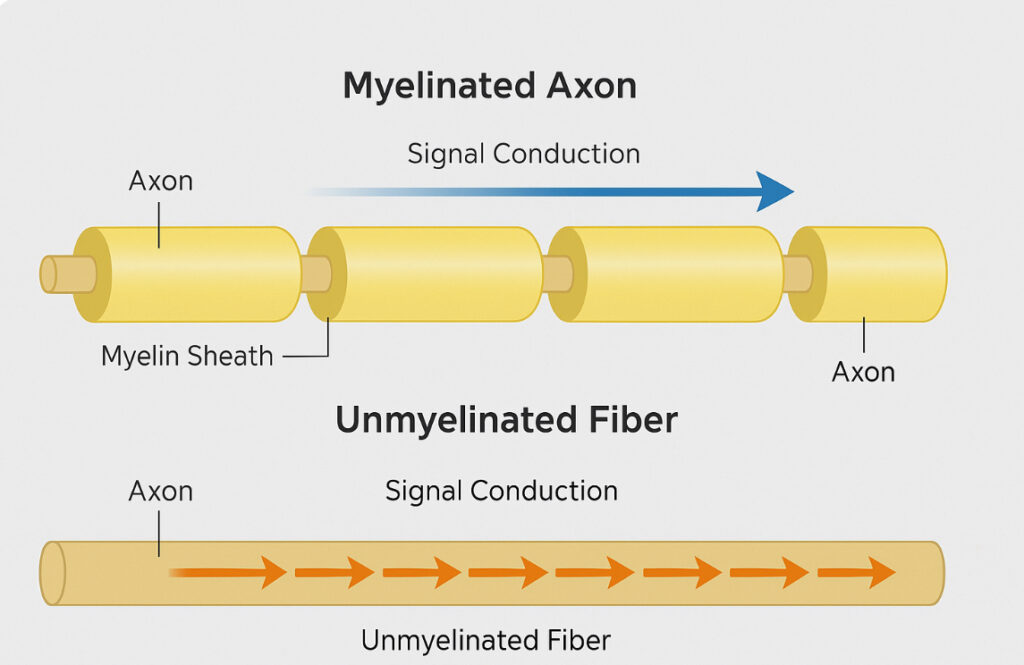
5. Integration in Brain Function
The collaboration between neurons, axons, myelin, and glial cells forms the foundation of
brain activity. Every thought, movement, emotion, and memory involves complex
interactions between these components.
Neurons initiate and propagate electrical signals. Axons carry these signals across long
distances, with myelin enhancing their speed and precision. Glial cells not only support
these processes structurally but also participate in chemical signaling, immune
responses, and synaptic modulation.
In sensory processing, for example, glial cells maintain the ionic balance required for
neuron firing, while myelinated axons transmit sensory data rapidly to the brain. In
cognition, astrocytes influence synaptic plasticity, which underlies learning and memory.Disruption in any part of this system-axon damage, myelin degradation, or glial
dysfunction-can result in profound neurological disorders, including Alzheimer’s disease,
epilepsy, and neuropathic pain.
6. Conclusion
The study of glial cells, myelin, and axons reveals a nuanced and interconnected view of
the nervous system. Far from being passive bystanders, glial cells are active agents in
maintaining and regulating neural activity. Myelin, by increasing conduction velocity and
reducing energy consumption, ensures that signals are transmitted with the speed and
fidelity necessary for complex behaviors. The axon, as the main conduit of neural
communication, ties together these functions.
As our understanding of these elements deepens, so too does our capacity to develop
treatments for neurological disorders that target not just neurons but the entire cellular
ecosystem of the brain. In the end, the nervous system is not just a network of neurons-it
is a symphony of cells, each playing a critical role in the concert of cognition consciousness.
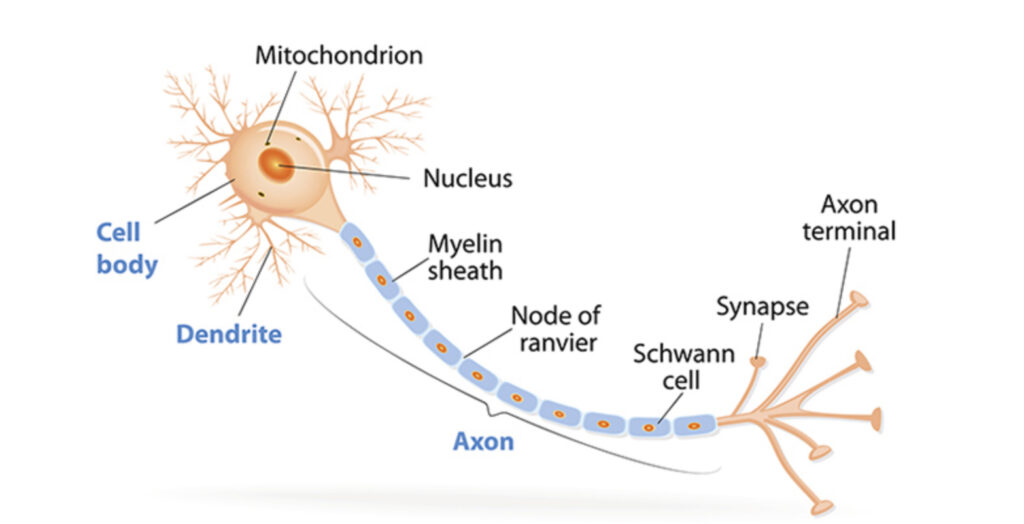